The Fructose, Uric Acid, and Evolution Conundrum: A Sweet Mystery
- S A
- Nov 11, 2024
- 8 min read
Fructose, a sugar commonly found in fruits and processed foods, affects the body in unique and potentially harmful ways, especially when consumed in excess. Unlike glucose, which is used by nearly every cell in the body for energy, fructose is primarily metabolised in the liver. This specialised metabolism has critical consequences that can lead to obesity and metabolic disorders. Let's examine the mechanisms by which fructose disrupts metabolic health.
The story of fructose and its metabolic pathway takes an unexpected turn when we consider uric acid. This blog (2), explores the evolutionary role of fructose, its conversion to uric acid, and the intriguing loss of the enzyme uricase in humans, leaving us with high uric acid levels compared to other primates.
The Evolutionary Perspective
Fructose has been a part of the human diet for millennia, primarily from fruits. From an evolutionary standpoint, fructose may have offered survival advantages by providing a rapid, concentrated source of energy, especially during periods when food was scarce. Hibernating animals like bears and migrating birds use this principle instinctively, gorging on fructose-rich fruits to prepare for seasons of scarcity. The fructose encourages fat storage, giving these animals a critical energy reserve that sustains them through long migrations or winter hibernation when food sources are unavailable. Similarly, early humans may have used fructose’s fat-storing potential as an adaptive survival response during periods of low food availability.
Fructose and Its Impact on Behaviour and Physiology
Stimulating Foraging Behavior:
Fructose can trigger a foraging response, characterised by increased exploratory behaviour, impulsivity, and locomotor activity. This behaviour may have been adaptive in the past, driving our ancestors to seek out food sources.
Fructose triggers behavioural changes that enhance the search for nourishment. It stimulates hunger and promotes bingeing behaviour via activation of orexin-hypothalamic circuits, while also induces thirst through increased serum osmolality linked to glycogen production.
Importantly, fructose disrupts normal weight regulation, impairing satiety and resulting in excessive energy intake over time due to central leptin resistance. This mechanism is critical for survival, encouraging foraging and exploratory behaviours.
Energy Conservation:
Fructose not only stimulates excessive caloric consumption but also promotes physiological adaptations like increased intestinal villi length for enhanced nutrient absorption. It facilitates lipogenesis while impairing fat oxidation and potentially reducing lipolysis due to hyperinsulinemia. These processes lead to higher glycogen and fat storage, ensuring energy reserves for times of scarcity.
Fructose can induce insulin resistance, leading to reduced glucose uptake by tissues like muscle and adipose tissue. This can help conserve energy for vital functions.
Fructose can also stimulate the production of uric acid, which can increase blood pressure and kidney function, potentially aiding in survival during periods of stress.
Although the foraging response necessitates energy expenditure, fructose aids energy conservation by reducing resting metabolic rates and impairing glucose uptake in muscle and adipose tissue. This preservation of blood glucose levels supports brain function, particularly in areas less reliant on insulin for glucose absorption, thus maintaining cognitive capabilities essential for foraging. However, this same insulin resistance affects self-control and decision-making, further promoting foraging behaviours while conserving energy.
An interesting side note: Camels are famous for their ability to go days without water, a feat linked to their humps. However, contrary to popular belief, their humps don’t store water directly. Instead, they store fat, which camels can metabolise for both energy and small amounts of water. When food and water are scarce, the fat in their humps serves as an energy reserve, breaking down into usable fuel and releasing water as a metabolic byproduct. This adaptation allows camels to endure arid conditions and long journeys across deserts.
This clever mechanism in camels resembles the way fructose may have once supported survival in humans and animals during times of scarcity.

Image Credit: https://doi.org/10.1098/rstb.2022.0230
The Loss of Uricase: A Missing Piece
During fructose breakdown in the liver, a byproduct called uric acid is produced. Uric acid is a by-product that the body typically excretes through urine. Most mammals possess the enzyme uricase, which efficiently breaks down uric acid into a more soluble form for easier excretion. However, humans lack a fully functional uricase gene:
The Evolutionary Puzzle: The exact reason for losing uricase is not fully understood. Some theories suggest it may have offered a survival advantage during times of scarce food:
Uric Acid as an Antioxidant: Uric acid may have acted as an antioxidant, potentially protecting early humans from free radical damage in times of low dietary intake.
Uric Acid and Vitamin C Synthesis: Uric acid may have played a role in vitamin C synthesis, which our bodies can no longer produce independently.
ATP Suppression and Metabolic Impact
Fructose uniquely disrupts energy regulation, independent of its caloric value, by inhibiting multiple cellular pathways. One such pathway is AMPK, a key energy sensor. When AMPK is suppressed, cells sense energy deprivation despite abundant nutrients, triggering increased hunger and fat storage. Fructose also inhibits beta-oxidation, halting fat-burning processes and channeling energy toward fat production instead.
Upon ingestion, the liver’s ATP may drop by up to 20% (and up to 70% if administered intravenously). This triggers a survival response where increased caloric intake is signalled. However, with mitochondria limited in function, much of this energy ends up stored as fat. Though ATP eventually replenishes, it does so at the cost of fat accumulation, ultimately elevating stored energy over usable energy and reinforcing metabolic dysfunction.
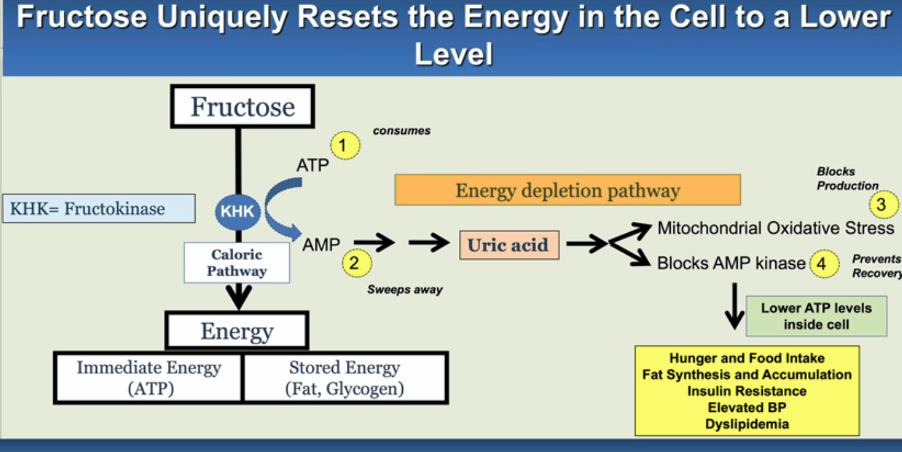
Image Credit: https://doi.org/10.1098/rstb.2022.0230
Fructose metabolism operates differently from glucose and has unique impacts on cellular biochemistry, especially in the liver:
AMPK Inhibition: Normally, AMPK (AMP-activated protein kinase) senses low energy, activating pathways to increase ATP production. Fructose metabolism suppresses AMPK, so cells interpret this as a signal of low energy, increasing hunger signals and promoting fat storage instead of energy utilisation.
In the AMPK Inhibition process, fructose metabolism rapidly depletes ATP due to the action of fructokinase-C (KHK-C), which converts fructose into fructose-1-phosphate. This enzyme operates without feedback regulation, causing a significant ATP drop and triggering AMP deaminase-2 (AMPD2) to convert AMP to IMP, which limits ATP regeneration and leads to uric acid production. Uric acid, alongside xanthine oxidoreductase, promotes oxidative stress in the mitochondria, impacting ATP generation and disrupting the citric acid cycle by inhibiting aconitase.
Beta-Oxidation Suppression: Beta-oxidation is the process of breaking down fatty acids for energy. Fructose, however, downregulates this process, which inhibits fat burning and directs energy toward fat synthesis (lipogenesis) rather than utilisation. Fructose metabolism disrupts beta-oxidation of fatty acids, by interfering with carnitine palmitoyltransferase-1α (CPT1α) and enoyl-CoA hydratase, essential components for transporting and metabolising fatty acids within mitochondria.
This interference limits fatty acid breakdown while fructose metabolites redirect energy processes towards fat storage instead of energy production. Furthermore, acetate produced from gut microbiota in response to fructose provides acetyl-CoA, fueling lipogenesis while bypassing ATP-generating beta-oxidation. This combination creates a metabolic environment favoring fat storage rather than fat burning.
Lack of Glycolysis Regulation: Glucose metabolism is tightly regulated by enzymes like PFK1 (phosphofructokinase-1), which controls glycolysis based on the cell's energy needs. Fructose metabolism bypasses this regulation, with fructose entering the glycolysis pathway past the point of PFK1 control. This means fructose is metabolised at an unchecked rate, regardless of cellular energy status.
GLUT2 Transporter and Unrestricted Entry: Fructose enters liver cells via the GLUT2 transporter, which does not rely on insulin and has a low affinity. This means that fructose can enter the liver in large quantities if it is abundant in the bloodstream, leading to an influx of fructose and its byproducts in the liver. Once inside, fructose is rapidly converted to intermediates that feed into fat synthesis pathways.
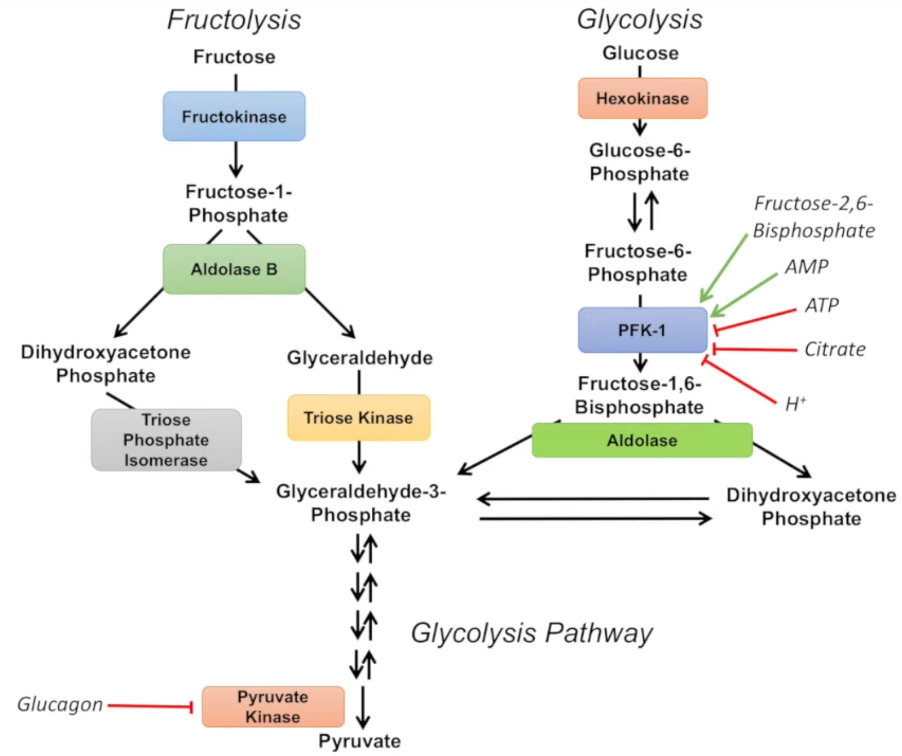
Image Credit: JJ Medicine
Here's a table highlighting key differences between fructose and glucose metabolism:
Aspect | Fructose Metabolism | Glucose Metabolism |
Pathway Location | Primarily in the liver | Occurs in various tissues, including liver and muscle |
Initial Enzyme | Fructokinase | Hexokinase or Glucokinase |
Regulation | Minimal regulation; bypasses key metabolic checkpoints | Highly regulated; phosphofructokinase is a control point |
Transport into Cells | Enters liver via GLUT2 (insulin-independent) | Enters cells via GLUT4 (insulin-dependent in muscle) |
Impact on ATP | Rapidly depletes ATP in the liver | Maintains ATP levels more stably |
Byproducts | Produces fructose-1-phosphate, uric acid, and promotes triglyceride synthesis | Converts to pyruvate and enters glycolysis for energy |
Insulin Response | No significant insulin release | Stimulates insulin secretion |
Storage Tendency | Encourages fat production and storage | Primarily used for immediate energy or glycogen storage |
Associated Effects | Increases uric acid, oxidative stress, and fat storage | Used for cellular energy without significant fat storage |
Adipogenesis and Fat Accumulation
When consumed chronically, fructose promotes the formation of white adipose tissue (WAT), or body fat, through a process known as adipogenesis. Fructose metabolism produces triglycerides and very-low-density lipoprotein (VLDL), both of which contribute to fat storage. The liver, overwhelmed by high fructose intake, often packages these fats into VLDL, which then circulate through the bloodstream and are stored in fat cells, leading to weight gain and an increased risk of obesity-related health issues.
Oxidative Stress
Another concerning aspect of fructose metabolism is its ability to increase oxidative stress in the liver. Oxidative stress occurs when there’s an imbalance between free radicals and antioxidants, leading to cellular damage. In the case of fructose, oxidative stress disrupts normal cellular function and favors fat storage over energy expenditure. This effect compounds the risk of obesity and associated metabolic diseases like insulin resistance and fatty liver disease.
The "Survival Switch"
Fructose also activates what scientists call a “survival switch.” In animals preparing for hibernation, high-fructose foods trigger mechanisms that promote energy storage for periods of scarcity. In humans, however, this survival response becomes problematic, especially with the constant availability of high-fructose foods. Frequent activation of this switch promotes ongoing fat storage and metabolic dysregulation, ultimately leading to a higher risk of obesity, diabetes, and other metabolic issues.
Overall, these combined effects lead to increased lipid production, reduced fat breakdown, and altered energy sensing—all contributing to fat accumulation, insulin resistance, and metabolic dysfunction. This biochemistry highlights why excessive fructose intake has been associated with obesity and metabolic disorders.
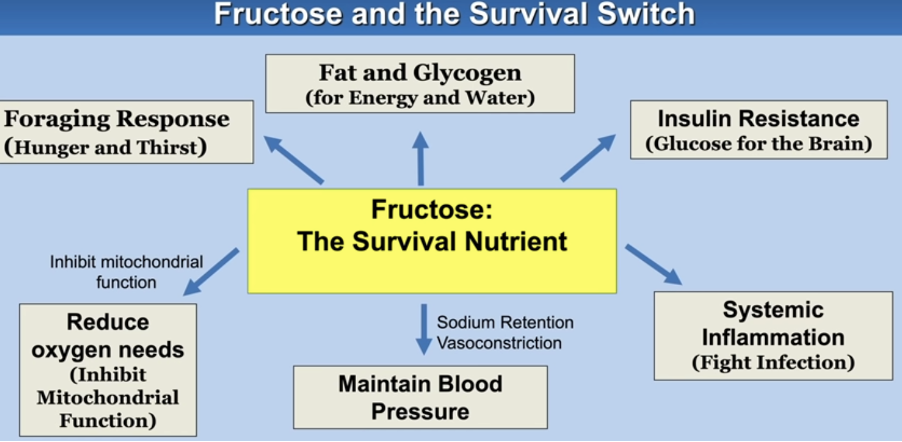
Image Credit: https://doi.org/10.1098/rstb.2022.0230
The Intriguing Debate:
The exact role of fructose, uric acid, and uricase loss in human evolution remains a topic of debate and ongoing research. Here are some additional points to consider:
Fructose Consumption and Uric Acid Levels: While fructose can contribute to uric acid production, other dietary factors like purines (found in red meat and organ meats to a lesser extent) also play a role. Purines from beer plays a major role in the production of uric acid.
Individual Variations: Not everyone who consumes high amounts of fructose develops hyperuricemia. Individual genetic predispositions may influence how the body handles uric acid.
Fructose: A Stealthy Contributor to Weight Gain
Fructose contributes to fat gain through several mechanisms that interplay to create an environment conducive to obesity. The dual effects of increased caloric intake and decreased resting energy expenditure are central to understanding how fructose drives weight gain.
Increased Energy Intake: Fructose stimulates a preference for sweet foods and can lead to heightened caloric consumption. Importantly, studies demonstrate that even in the absence of taste perception, animals still favor fructose, highlighting its metabolic effects rather than just sensory pleasure. The development of leptin resistance plays a crucial role here. Initially, fructose consumption reduces chow intake, but over time, it leads to increased food intake due to a compromised signalling of satiety, resulting in hypercaloric consumption and subsequent weight gain.
Reduced Resting Energy Metabolism: Fructose decreases resting energy expenditure, which can contribute to weight gain when combined with increased caloric intake. Animal studies show that those fed fructose exhibit minimal weight changes in the short term, suggesting that the long-term effects on body composition are more pronounced and linked to these metabolic adaptations.
Leptin Resistance: Chronic fructose consumption leads to central leptin resistance, a condition where the brain becomes less responsive to leptin, the hormone responsible for regulating energy balance and appetite. This resistance is associated with a shift in energy storage mechanisms, pushing excess calories toward fat accumulation rather than being utilised for immediate energy needs.
Evolutionary Perspective: Fructose may have evolved as an adaptive mechanism to enhance survival during times of food scarcity by promoting fat storage and increasing foraging behavior. This survival switch, while beneficial in ancient environments, becomes detrimental in modern contexts where high-fructose diets are prevalent.
The interplay of these mechanisms illustrates how fructose metabolism can lead to fat gain, driving obesity and its associated metabolic disorders.
Conclusion
The metabolism of fructose plays a significant role in the development of obesity and metabolic disorders. By suppressing ATP levels, inducing leptin resistance, and shifting energy utilisation towards fat storage, fructose not only increases caloric intake but also reduces energy expenditure. This complex interplay highlights the evolutionary origins of fructose as a survival mechanism, which, in today's high-fructose diet context, leads to detrimental health outcomes. Understanding these mechanisms can inform dietary choices and strategies for combating obesity and related metabolic issues.
By understanding these impacts, individuals can make informed dietary choices, prioritising nutrient-dense foods and limiting excessive fructose intake to support better long-term metabolic health.
Comments